The serial mechanical exfoliation method available can not be used for the assembly of graphene in the large scale. In this process, deposition of ultrathin few-layer (three to ten) graphene oxide, with parallel and controllable assembly, by dielectrophoresis between prefabricated electrodes has been demonstrated.
Brian R. Burg, Fabian Lütolf, Julian Schneider, Niklas C. Schirmer, Timo Schwamb, Dimos Poulikakos
Laboratory of Thermodynamics in Emerging Technologies, ETH Zurich, 8092 Zurich, Switzerland
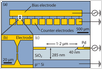
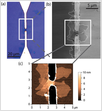
1 g of natural graphite flake is mixed for 5 days in a 62.1 ml solution of concentrated H2SO4 containing 0.75 g NaNO3 and 4.5 g KMnO4.
Then it is washed in 100 ml 5 wt % H2SO4 and reacted with 3 g of 30 wt % H2O2 in H2O.
Impurities are removed by multiple (15×) centrifugation and ultrasonic precipitate re suspension in aqueous 3 wt % H2SO4 and 0.5 wt % H2O2.
After washing the solution with H2O on a cellulose suction filter 16–40 µm pore size and letting the dispersion rest for 1 day, a last centrifugation cycle (8×) in H2O is performed with the supernatant half of the solution.
After drying the single-layer particles, they are ultrasonically redispersed in water at a concentration of 1 mg/ml.
A droplet (5µl) of the aqueous GO solution is dispensed on the chip, and a sinusoidal potential difference is applied to the bias electrode.
After 60 s the generator is switched off, the droplet is blown off by a stream of nitrogen gas, and the is chip rinsed in de-ionized water.
The sample is characterized by light microscopy, scanning electron microscopy SEM, atomic force microscopy AFM, and electric transport measurements.
- Thermal annealing of the samples is performed in a rapid thermal anneal oven J.I.P. Elec JetFirst 100 in an inert N2 environment.
- Reduction in the FLGO thin lms is confirmed by a sheet conductivity increase and decrease in layer thickness.
- 1 g of natural graphite flake
- 62.1 ml solution of concentrated H2SO4 containing 0.75 g NaNO3 and 4.5 g KMnO4
- 100 ml 5 wt % H2SO4
- 3 g of 30 wt % H2O2 in H2O
- exfoliated graphene oxide
- Centrifuge
- Ultrasonic
- function generator
- scanning electron microscopy
- atomic force microscopy